Research Areas
Sensory Feedback
Neuromorphic Encoding
In order to naturalistically convey sensory information to amputees, we need to communicate with the nervous system using its own language. Neurons communicate through the generation and propagation of all-or-nothing action potentials (also referred to as spikes) that encode information or commands. Spiking activity can be induced through the use of electric stimulation. We seek to encode sensory information (such as touch or pain) as electrical stimulation pulses that will restore natural sensory perception for amputees.
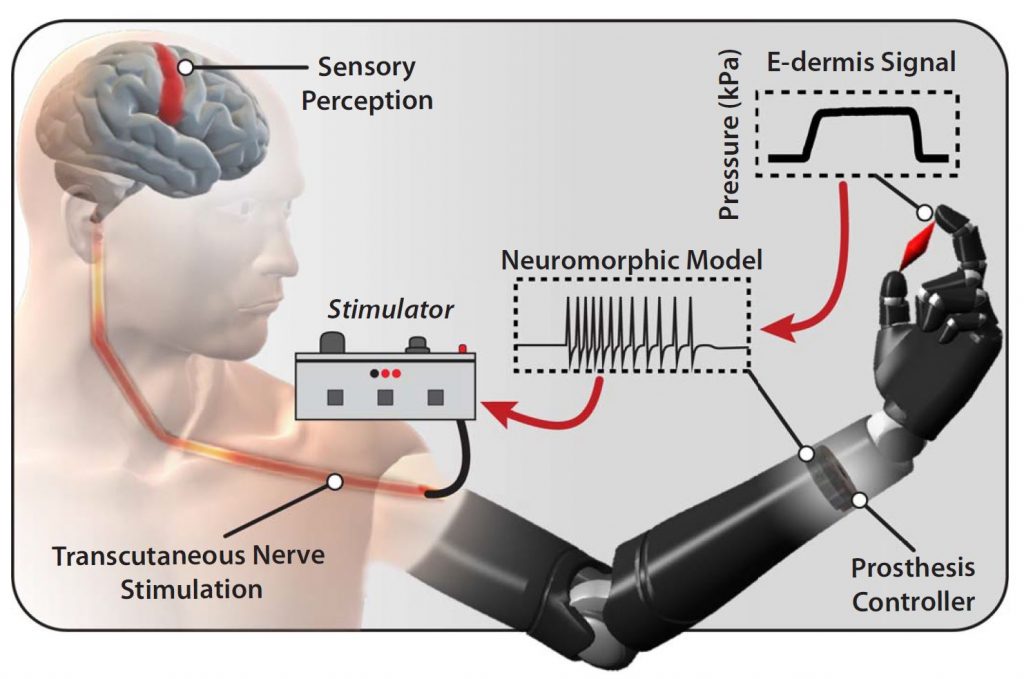
This encoding is called “neuromorphic” because it takes inspiration from the information processing pathways of the nervous system. Neuromorphic encoding of sensory information can be incorporated in several ways: in the design of the sensors themselves, in the multiplexing and compression of sensory information streams into fault-tolerant and noise-robust representations that are time-, space-, and energy-efficient, and in the extraction of high-level abstract information such as texture or shape. In addition to enabling closed-loop naturalistic sensory feedback systems for amputees, neuromorphic encoding may lead to more robust and efficient hardware and algorithms for general computing systems.
Cortical Processing
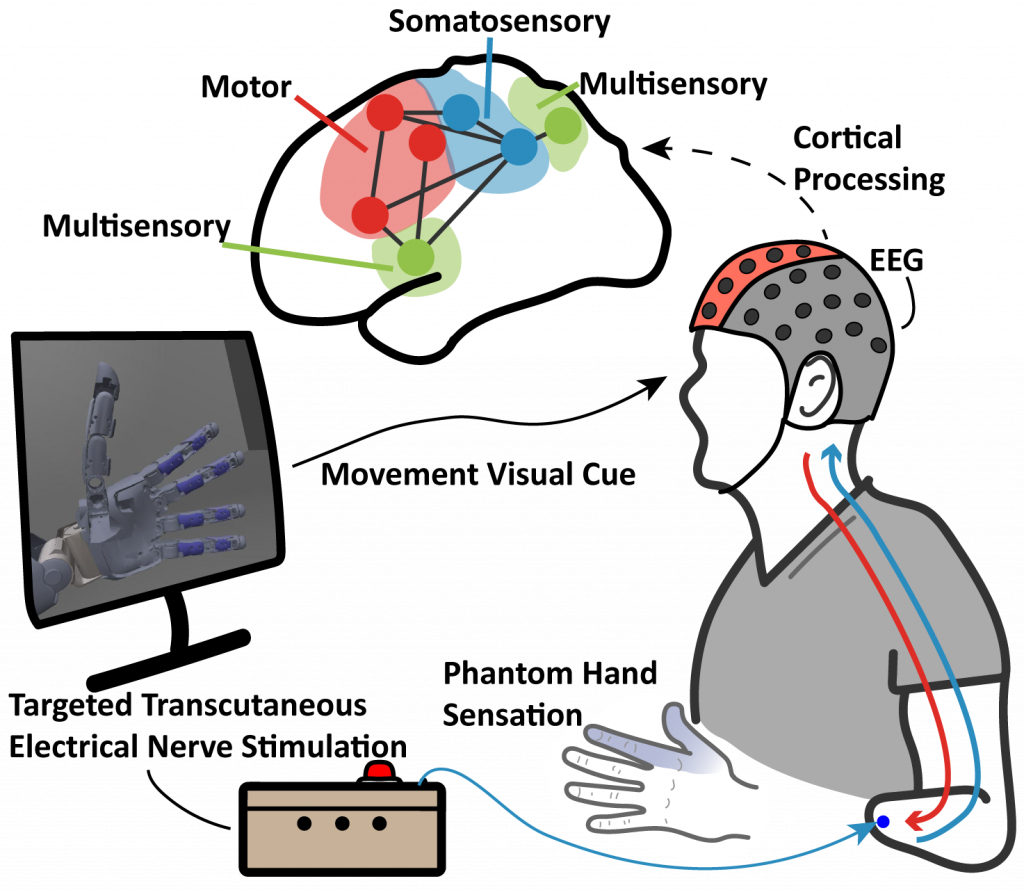
How does the brain, especially of individuals with an amputation, response, perceive and process sensory feedback signals? We use electroencephalogram (EEG) to characterize the neural activities and changes in response to sensory stimulation, through methods such as event related potentials (ERP) and functional connectivity. By modeling the cortex as a dynamic functional network, we can identify the mechanistic and temporal changes of the brain’s functional systems. This approach provides insights into the cortical impact of sensory stimulation with prosthesis movement and future paradigms for developing sensory restoration modalities and personalized neuroprostheses.
High Density Sensing and Neuromorphic Feedback
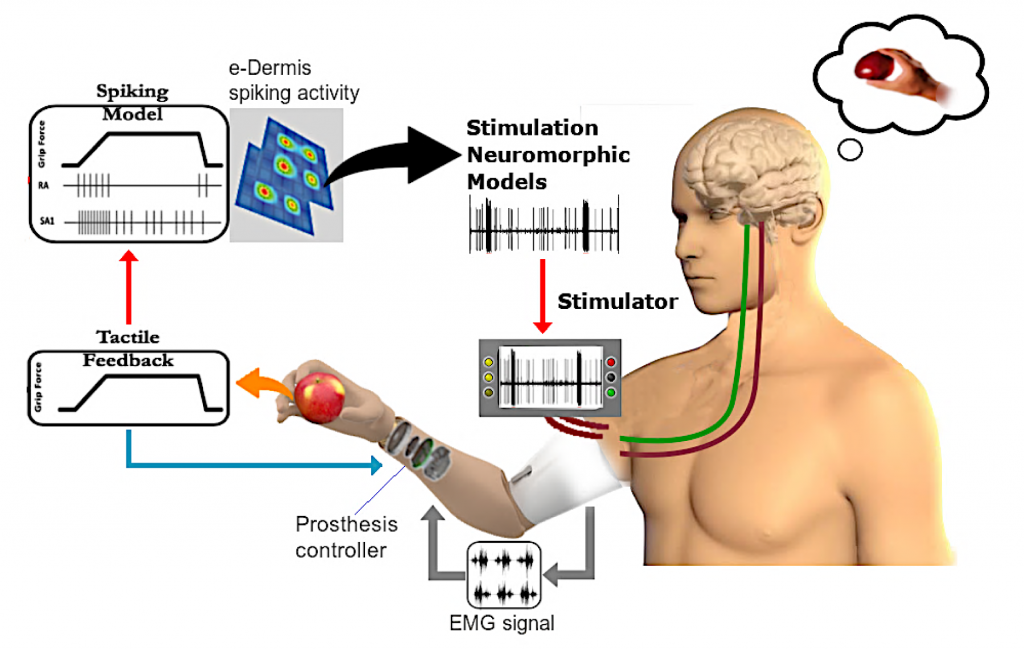
The goal of this project is to improve current upper-limb prosthesis by providing sensory perception through a closed-sensorimotor-loop using high-density tactile sensing with multichannel neuromorphic feedback. Moreover, the enhanced perception rendered by our approach will facilitate prosthesis embodiment and improve dexterous motor control and satisfaction with the prosthesis, leading to consistent use in daily activities and productive return to work.
Multimodal Feedback
We focus on non-invasive strategies to convey sensory information to users. The stimulation modalities we use are transcutaneous electrical nerve stimulation and vibratory stimulation. Electrical feedback elicits phantom sensations in amputees. Haptic feedback using vibration can be applied in human-machine interactions. We use various experiments and techniques such as psychophysics and EEG recordings to assess the functionality of each stimulation modality.
Motor Control
Myographic Decoding
Electromyography (EMG) is the measurement of electrical signals generated by muscles in response to stimulation by motor neurons, and can be used to gain understanding of motor intent. Surface EMG utilizes electrodes placed on the skin to collect these readings, and is a popular choice of control signal for upper limb prosthesis, due to their relatively large amplitude and ease of data acquisition. There are multiple paradigms for translating EMG signals into commands for prosthesis control, including ‘pattern recognition’, which uses machine learning techniques for either discrete classification or regression decoding in a continuous workspace. We are interested in advanced decoding techniques capable of achieve dexterous control, and adaptations to make these methods robust enough for use in clinical settings. We are also investigating the use of High Density electrode arrays to help create more robust systems.
Optimal Control
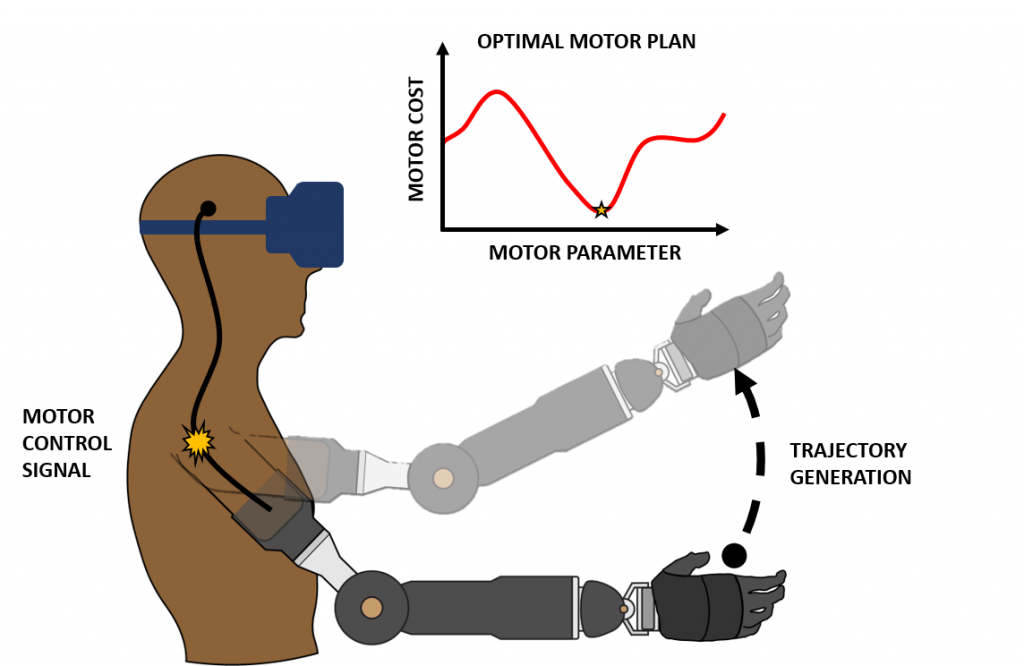
To dexterously interact with objects in the real world, the brain must compute an optimal motor control plan amongst an infinitely large set of possible movements. For someone with an intact limb, it is known that various factors, such as motor kinematics, dynamics, and energetics play a role in determining what type of motor plan is considered “optimal”. This computation is further complicated for an individual with limb loss because there is often unreliable forward control of their prosthetic device coupled with fundamental differences in their musculoskeletal system and an incomplete internal model of the residual limb-prosthesis system. The goals of this project are then (1) to determine the parameters of movement that cause an amputee to prefer one motor plan over another and (2) to explore interventions that induce able-bodied motor plans as optimal solutions for amputees as well.
Systems and Interfaces
Augmented/Virtual Reality
Traditional rehabilitation environments for upper limb amputees are costly in time, space, and money as they require the constant cooperation of a highly skilled clinician. Unfortunately, this requirement often limits the rate at which an amputee can acclimate to and improve the performance of their prosthetic device. The goal of this project is to develop training and evaluation environments in augmented and virtual reality for upper limb amputee use. In these environments, an amputee controls a prosthetic limb to manipulate virtual objects using a variety of control strategies and feedback modalities. It is the hope that motor skills learned in these virtual object relocation tasks result in improved real-world performance of the physical prosthesis.
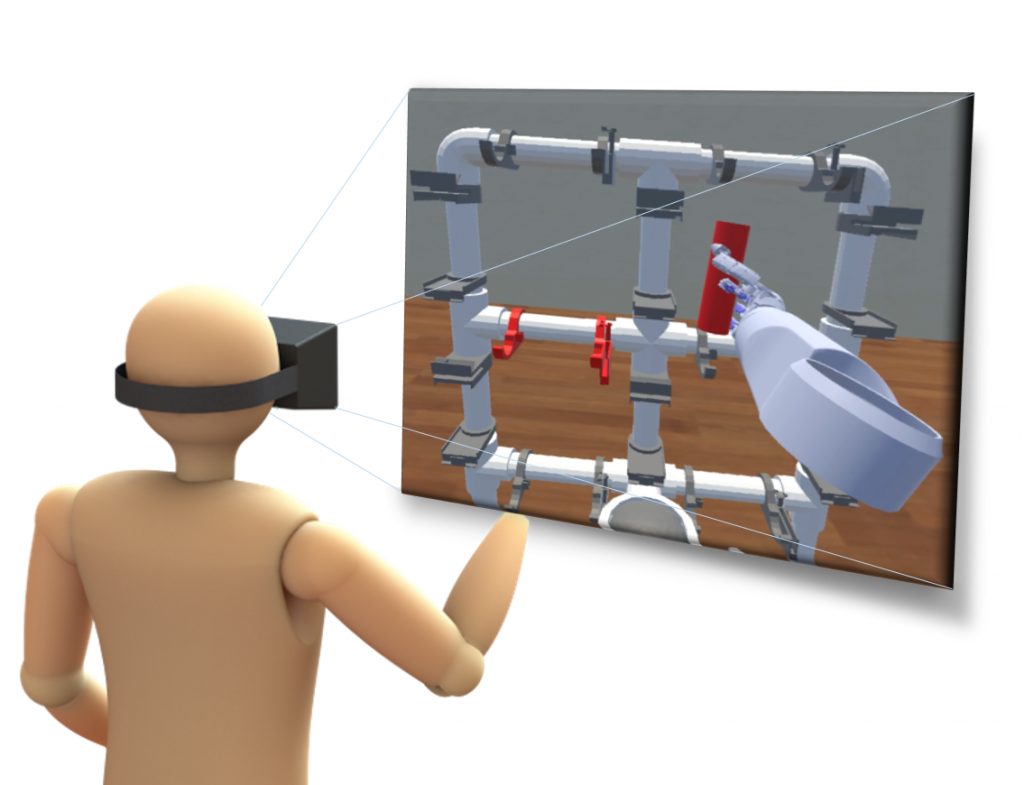
Soft Prosthesis
The compliant nature of soft robotic fingers allows for safe and dexterous manipulation of objects in an unstructured environment. Additionally, texture information can be essential to properly manipulate objects. Therefore, a soft biomimetic finger with texture discrimination feedback has the potential to enhance user experience when interacting with their surroundings.
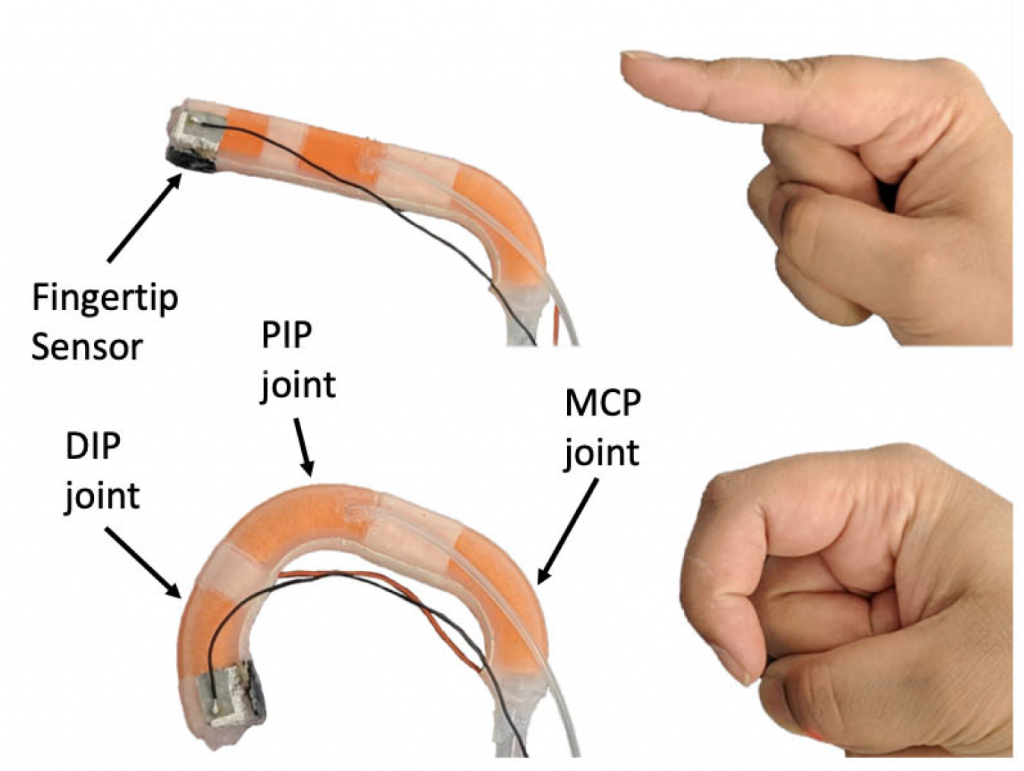
Muscle Reinnervation
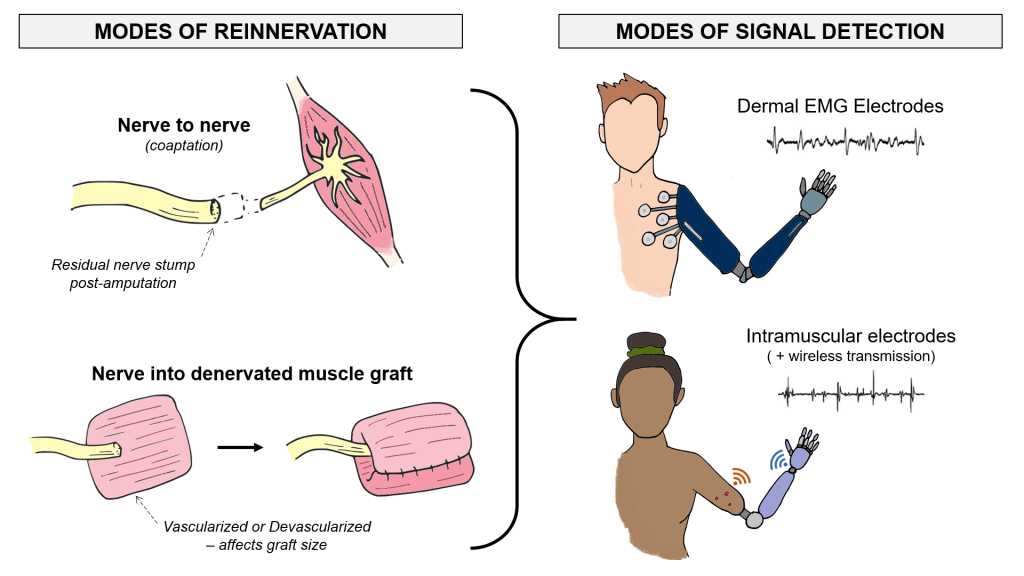
Amputated nerves are still capable of sending electrical control signals from the brain. That is why many amputees receive reconstructive surgeries to find new muscle targets for their damaged nerves (e.g., nerve coaptation, muscle graft interface, etc.). Our research on this process of reinnervation characterizes the different bio-electrical properties of the reinnervated muscles in amputees who receive different surgical interventions. Addressing the wide anatomical variation among the upper-limb amputee population will give us a better ability to design custom myoelectric prostheses. Additionally, we can investigate which surgical interventions give amputees the best electrophysiology for intuitively controlling a prosthetic hand.
Adaptive Socket
The human-machine interface in a prosthesis is a socket which connects to the residual limb. However, sockets have remained rigid and uncomfortable, leading users to abandon the device and leaving the technology underutilized. Our project aims to improve the interface by investigating the prosthetic socket’s loading effects on the amputated limb during static and dynamic use cases. To address the loading in a socket, we are investigating the use of soft robotic materials and actuators to evenly distribute and correct any load in a prosthetic socket due to daily use.
Clinical Neuroengineering
Cerebrovascular Autoregulation
Our laboratory has the privilege of working both at the Johns Hopkins Homewood campus as well as at the Johns Hopkins School of Medicine in East Baltimore. This connection to the hospital helps to foster clinical collaborations, particularly with Romer Geocadin, MD and Sung-Min Cho, DO, MHS in the Department of Neurology. Together, our labs work to investigate neuroprotective strategies that can be employed during/after cardiac arrest.
Under normal conditions, the cardiovascular system undergoes adjustments and changes to ensure that the brain receives the large amounts of oxygen it requires for functioning. During cardiac arrest, this important balance becomes impossible to maintain as blood flow is halted, which can cause long-lasting brain damage and loss of consciousness, such as a coma. Our lab has performed rodent studies to demonstrate how administration of Orexin-A can reduce neuroinflammation, and how therapeutic hypothermia can promote cerebral blood flow recovery.
In addition to our collaborations with Neurology, a strong collaboration with Prof. Arvind Pathak’s lab in Radiology has provided us the technology and expertise necessary to monitor cerebral blood flow with cutting-edge imaging techniques.
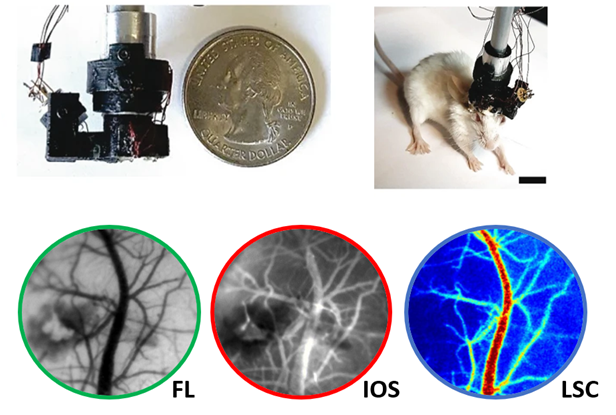
Ultrasound Stimulation
While much of the neurostimulation our laboratory employs is electrical, ultrasound neurostimulation is an exciting new area of research. We are collaborating with Amir Manbachi, MSc, PhD, in the Department of Neurosurgery in order to investigate how ultrasound can be used to stimulate neurons in the brain as well as to stimulate cerebral blood flow. (More exciting updates to come in the future!)
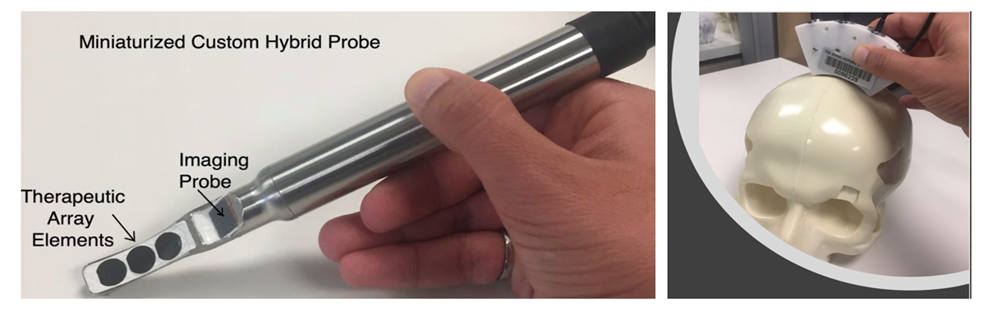